How to Address the Challenges of Tension Control for Advanced New Materials
Table of Contents
Advanced new materials, often characterized by their unique properties such as high strength-to-weight ratios, enhanced thermal stability, and excellent flexibility, present new challenges in manufacturing and processing. Among these challenges, tension control emerges as a critical factor in ensuring the quality, reliability, and performance of products made from these materials.
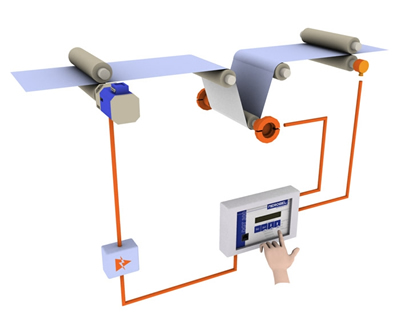
Understanding Tension Control in Material Processing
Tension control refers to the regulation of the tensile force applied to materials during manufacturing processes such as rolling, drawing, printing, and coating. Proper tension control is vital to maintaining the integrity of the material, ensuring consistent thickness, preventing defects like wrinkles, stretching, or breakage, and achieving the desired mechanical and physical properties in the final product.
In traditional materials like steel or conventional polymers, tension control is relatively straightforward, as these materials have well-understood properties and predictable behavior under stress. However, advanced new materials, such as carbon-fiber composites, shape-memory alloys, and high-performance polymers, exhibit complex behaviors that challenge conventional tension control techniques.
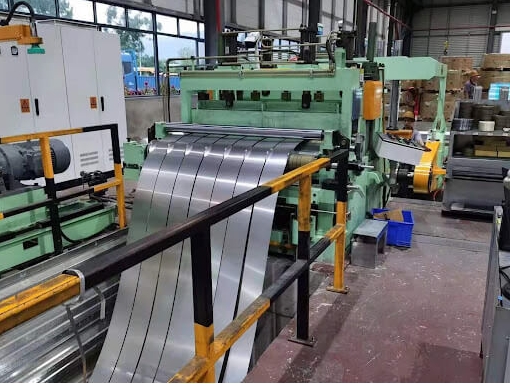
Challenges in Tension Control for Advanced New Materials
1. Material Heterogeneity and Anisotropy
- Definition: Advanced materials, such as carbon-fiber composites, often have heterogeneous (non-uniform) and anisotropic (direction-dependent) structures. This means that their mechanical properties can vary significantly in different directions.
- Challenge: Traditional tension control systems are designed for isotropic materials, where properties are uniform in all directions. The anisotropy in advanced materials requires customized tension control that accounts for varying strengths and stiffnesses along different axes.
2. Sensitivity to Environmental Conditions
- Definition: Many advanced materials are highly sensitive to environmental factors like temperature, humidity, and chemical exposure.
- Challenge: For instance, shape-memory alloys change their mechanical properties with temperature variations. This sensitivity demands real-time adjustments to tension control to maintain material integrity, as any environmental change can significantly affect the material’s behavior under stress.
3. Nonlinear Stress-Strain Behavior
- Definition: Unlike traditional materials, which often exhibit a predictable linear relationship between stress and strain, advanced materials may display nonlinear, time-dependent behaviors such as viscoelasticity or plasticity.
- Challenge: This nonlinearity complicates tension control, as small changes in applied stress can result in disproportionate changes in strain. Predicting and controlling the material’s response under these conditions requires sophisticated models and control systems.
4. High Precision Requirements
- Definition: Advanced materials are often used in high-stakes applications, such as aerospace, medical devices, and electronics, where precision is paramount.
- Challenge: Even minor deviations in tension can lead to defects like micro-cracks, delamination, or surface imperfections, which could compromise the final product’s functionality or safety. Achieving the required precision in tension control necessitates cutting-edge systems capable of maintaining tight tolerances.
5. Complex Manufacturing Processes
- Definition: The processing of advanced materials often involves complex, multi-stage manufacturing techniques, such as layering, resin infusion, or high-temperature curing.
- Challenge: Each stage of the manufacturing process may have different tension control requirements. For example, during the production of composite materials, tension must be carefully managed during fiber placement, resin application, and curing to avoid defects. Coordinating tension across these various stages adds complexity to the control process.
6. Dynamic Material Behavior
- Definition: Some advanced materials exhibit dynamic behavior, where their properties change during the manufacturing process, such as phase changes or chemical reactions.
- Challenge: Managing tension in materials that change state or properties during processing requires real-time monitoring and adaptive control systems that can respond to these dynamic changes without causing material damage or defects.
These challenges highlight the need for advanced tension control systems that can accommodate the unique properties and processing requirements of new materials, ensuring consistent quality and performance in the final products.
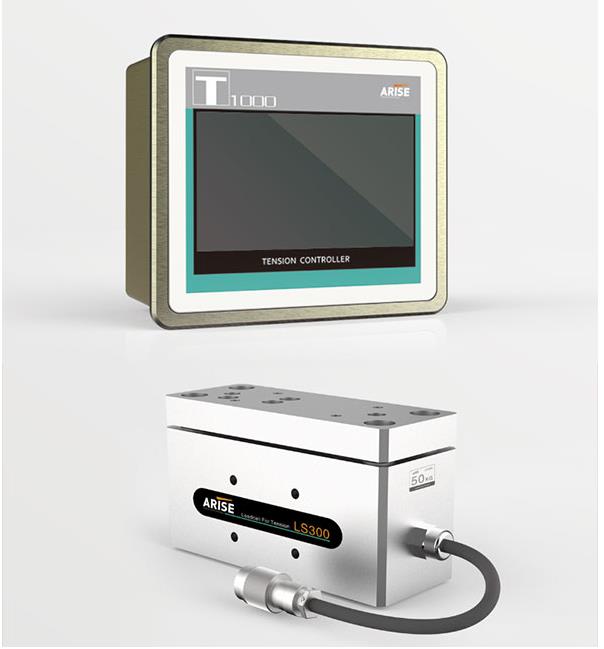
Solutions and Innovations in Tension Control for Advanced New Materials
1. Advanced Tension Control Systems
- Solution: Leveraging digital technologies like real-time sensors, automated feedback loops, and machine learning algorithms, modern tension control systems offer precise regulation of tensile forces during material processing.
- Innovation: These systems can dynamically adjust tension based on real-time data, responding to changes in material properties, environmental conditions, and processing speeds. This adaptability ensures consistent quality, even with the complex behaviors of advanced materials.
2. Adaptive and Predictive Control
- Solution: Adaptive control systems can modify their operation in response to the material’s behavior during processing, while predictive control systems use advanced modeling to anticipate changes in material properties.
- Innovation: By integrating predictive algorithms that forecast how materials will react to tension, these systems can make preemptive adjustments, ensuring that tension is maintained within optimal ranges. This approach is particularly effective for managing nonlinear and time-dependent behaviors, such as those seen in viscoelastic or shape-memory materials.
3. Integrated Process Control
- Solution: Tension control systems can be integrated with other process parameters, such as temperature, pressure, and speed, to create a holistic approach to material processing.
- Innovation: In processes like composite manufacturing, where multiple stages such as fiber placement, resin infusion, and curing are involved, coordinating tension control with these parameters can reduce defects. For example, synchronizing tension adjustments with resin flow rates can ensure uniform distribution and reduce the risk of delamination.
4. Material-Specific Control Strategies
- Solution: Developing tension control strategies tailored to the specific characteristics of each advanced material can significantly enhance processing outcomes.
- Innovation: For anisotropic materials, control systems can be designed to apply different tensions in different directions, ensuring that the material’s unique properties are preserved and optimized. Custom tension control algorithms can also account for the specific stress-strain curves of advanced materials, leading to more precise control.
5. Real-Time Monitoring and Feedback
- Solution: Implementing real-time monitoring systems that continuously measure material tension and related parameters during processing can provide immediate feedback to control systems.
- Innovation: By utilizing high-resolution tension sensors and advanced data analytics, these systems can detect minute changes in tension and make instant corrections. This real-time feedback loop is critical for maintaining the tight tolerances required in the processing of advanced materials, especially in applications like aerospace or medical devices.
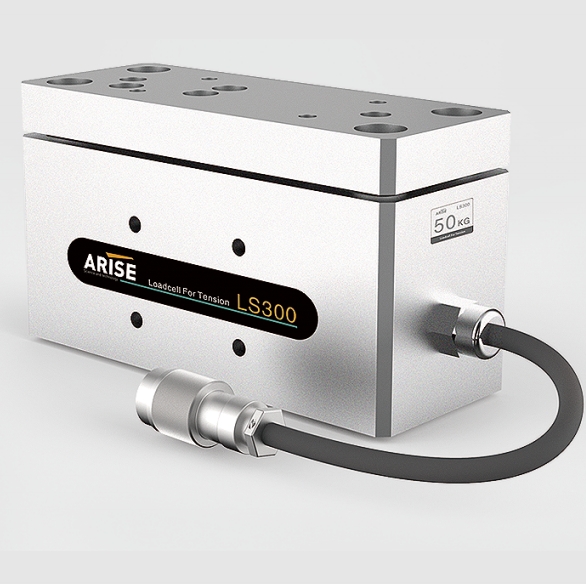
6. Smart Materials and Self-Regulating Systems
- Solution: The development of smart materials that can self-regulate their tension or provide feedback on their stress state is an emerging area of innovation.
- Innovation: These materials could incorporate embedded sensors or actuators that automatically adjust tension in response to changes in environmental conditions or processing stages. For example, a composite material with integrated piezoelectric elements could adjust its tension in real-time, reducing the need for external control systems.
7. Simulation and Digital Twins
- Solution: Using simulation tools and digital twin technologies to model and predict tension control scenarios before actual production begins.
- Innovation: Digital twins create a virtual replica of the material and the processing environment, allowing for the testing and optimization of tension control strategies in a risk-free virtual setting. This predictive capability helps identify potential issues and fine-tune control systems, leading to more efficient and accurate production runs.
Overcoming the challenges posed by advanced materials in tension control requires innovative control systems and strategies. As industries increasingly adopt these materials, the development and implementation of such advanced tension control strategies will be key to achieving high-quality and efficient manufacturing processes.